exoplanets
All posts tagged exoplanets
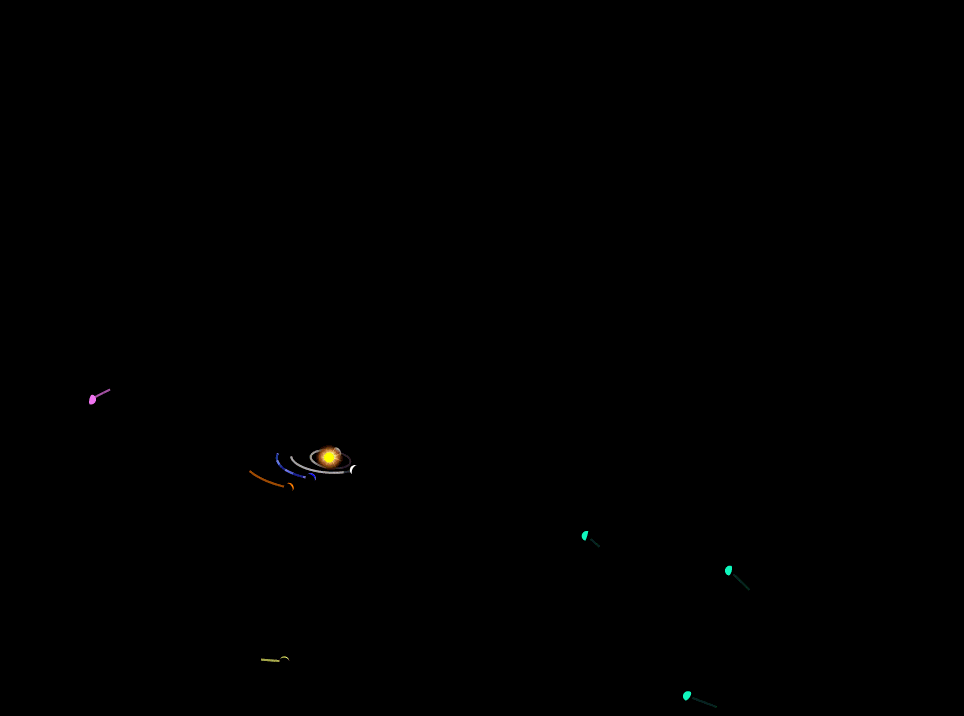
Nominal trajectory of ‘Oumuamua. From https://en.wikipedia.org/wiki/File:C2017U1.gif.
A month ago, astronomers found, for the first time, an asteroid that definitely originated from outside our solar system.
The object, 1I/ʻOumuamua, came screaming into our solar system at 60,000 mph, took a sharp turn around the Sun, and passed within 10 million miles of Earth on Oct 18 before beginning its long journey out of our solar system and back into interstellar space.
Given its highly elongated and inclined orbit, ʻOumuamua was initially classified as a comet, but follow-up observations showed no sign of a coma, and so it was re-classified as an asteroid. Its discovery has prompted a flurry of short but exciting astronomical studies, and in our research group meeting this week, we discussed two: Ye et al. (2017) and Laughlin & Batygin (2017).
In their study, Ye and colleagues describe their observations of ʻOumuamua’s brightness and color. Their color observations indicated that ʻOumuamua is slightly but not very red, unlike many icy bodies in our Kuiper Belt. This result suggests it either formed close to its original central star (and never had much ice) or spent time near enough to its original parent star to have baked off any ice.
They also estimated that ʻOumuamua passed very near Earth’s orbit, close enough that, if any material were ejected from its surface, it may produce a meteor shower in a few hundred years.
In their study, Laughlin and Batygin took a more theoretical tact and explored possible implications of ʻOumuamua’s for the existence of planets like the putative Planet Nine.
ʻOumuamua almost definitely originated in a distant solar system and was ejected by a gravitational interaction with a planet in that system, and Laughlin and Batygin point out that most of the known exoplanet population would probably not be very good at ejecting objects like ʻOumuamua: these planets are so small and/or close to their host stars that they cannot easily liberate asteroids like ʻOumuamua from the host stars’ gravitational clutches.
But, Laughlin and Batygin suggest, if there is a sizable population of largish (several Earth masses) planets several times farther from their host stars than Earth is from the Sun, then gravitational ejections of asteroids might occur frequently enough to explain objects like ʻOumuamua.
Granted, they’re dealing with a sample size of one, but several all-sky surveys, like LSST and TESS, will arrive on the scene any day now. And we may very soon find other interstellar interlopers like ʻOumuamua. The galaxy is probably full of them.
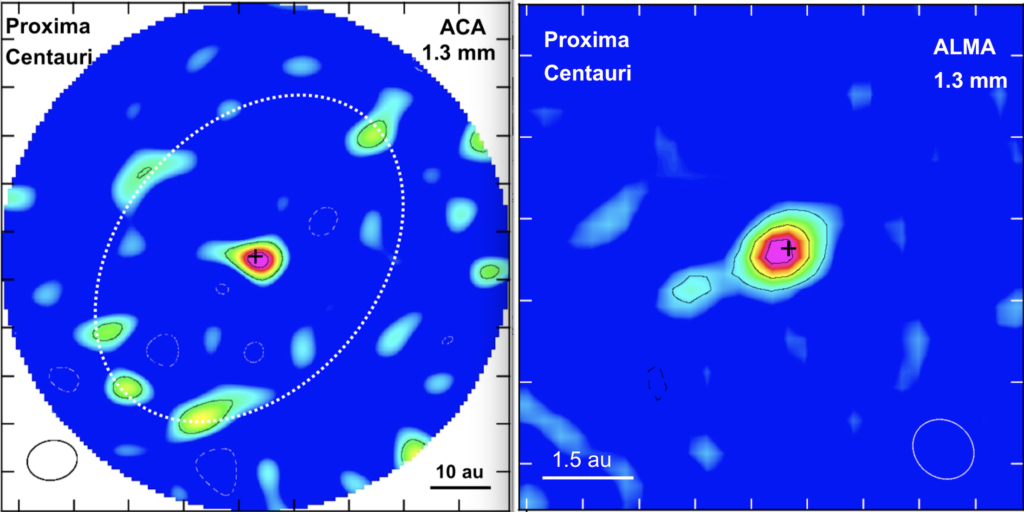
Figure 1 (left) and 2 (right) from Anglada et al. (2017). The right figure shows a zoomed-in version of the left figure. The rainbow blob at the center of the left figure is Proxima Centauri’s debris disk, and the white ellipse shows the possible outer disk. The greenish blob just to the left of center in the right figure is the mysterious source, possibly a ringed planet.
In case you didn’t hear, late last year, astronomers confirmed a planet around our nearest stellar neighbor, Proxima Centauri, a red-dwarf star just four light years from Earth. The planet is probably about 30% more massive than Earth, probably making its composition Earth-like, and it’s in the habitable zone of its star, at a distance of about 0.05 astronomical units (AU) – all of which make it an exciting prospect for follow-up studies.
And just last week, Guillem Anglada and colleagues announced the further discovery of a debris disk around the star. The left figure up top shows the image, in radio wavelengths, of emission from the disk – the disk appears as the rainbow blob near the center, and the location of the host star Proxima is marked with a black cross.
The disk’s appears to orbit between 1 and 4 AU from its host star, which would put it between the Earth and Jupiter if it orbited in our solar system. However, since the red-dwarf star is so much smaller and cooler than our Sun, those orbital distances correspond to temperatures of only a few tens of degrees, making Proxima’s disk more akin to our Kuiper belt than our main asteroid belt.
The radio light we see from the disk is mostly due to thermal emission from dust. Using the above temperature estimate (and some other reasonable assumptions), Anglada and colleagues estimate (with large uncertainties) Proxima’s disk has about one thirtieth the mass of Ceres in dust and a lunar mass in larger bodies – almost as much mass as our Kuiper belt. There’s also marginal evidence in the data for a larger and cooler disk as well, perhaps 30 times farther from the star than the inner disk, and for something perhaps even more interesting.
In the right figure above, see the greenish blob just below and to left of the rainbow blob? That (admittedly weak) signal could be emission from a ring system orbiting a roughly Saturn-mass planet about 1.6 AU distant from the star. The authors point out that there’s a small but non-zero chance that it’s actually just a background galaxy that photobombed their observations, a possibility that can be easily tested by looking at Proxima again in a few months. But if it turns out to be a ringed planet, it would be the first exo-ring system directly imaged (other systems show possible signs of rings).
That would make Proxima an even more unusual planetary system since small stars tend to have small planets, and I’m only familiar with one other red dwarf star that hosts a big planet – NGTS-1 b, a red-dwarf hosting a hot Jupiter. But if there’s one thing that exoplanet astronomy has taught us in the last few decades, it’s to expect the unexpected.
The diagram below shows the structure of the Proxima Centauri system suggested by Anglada and colleagues.
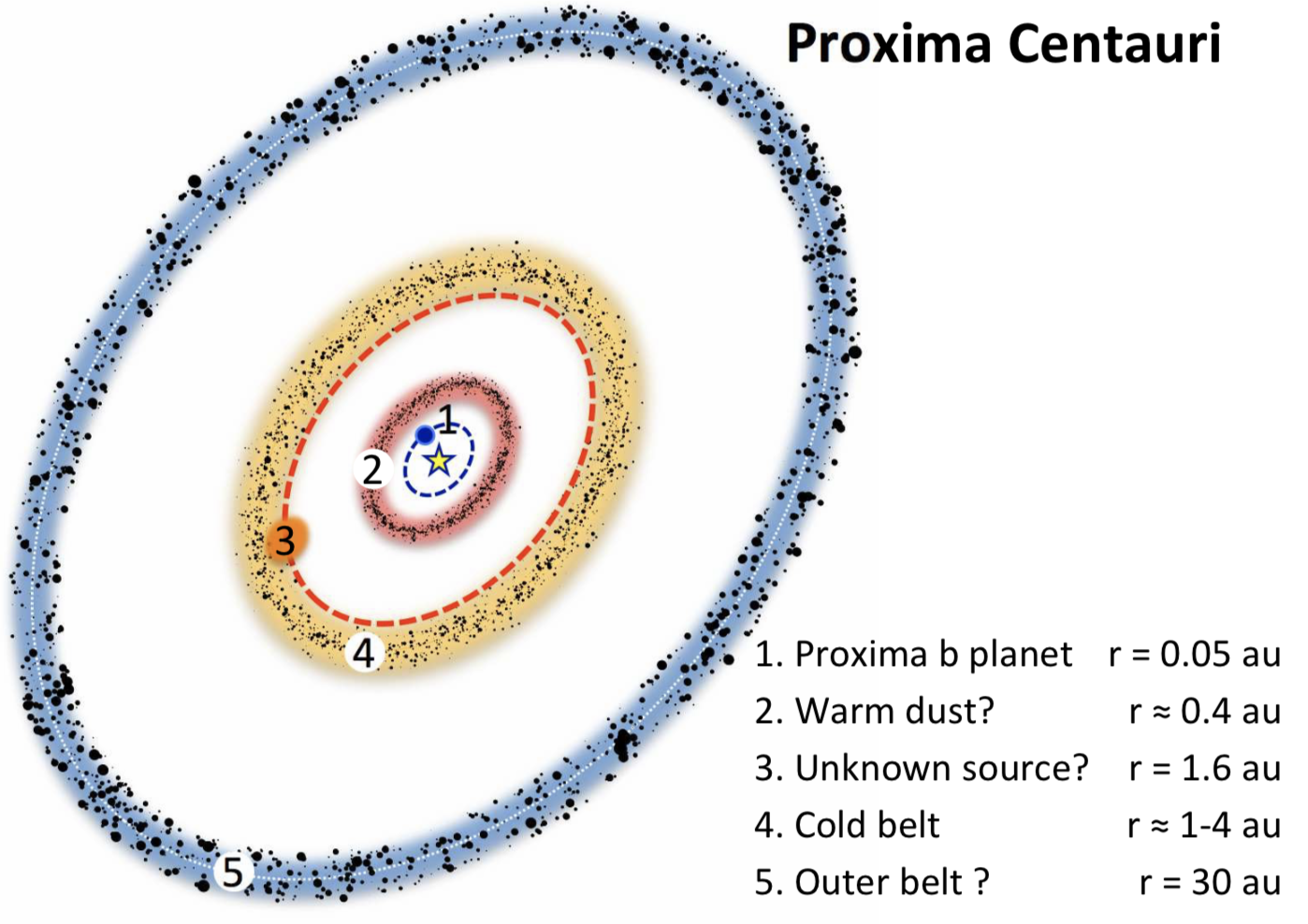
Figure 4 from Anglada et al. (2017), showing the suggested structure of the Proxima Centauri planet-disk system.
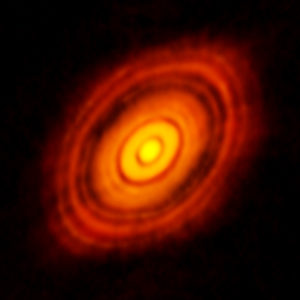
This is the sharpest image ever taken by ALMA — sharper than is routinely achieved in visible light with the NASA/ESA Hubble Space Telescope. It shows the protoplanetary disc surrounding the young star HL Tauri.
Exoplanets are being discovered from near and far, and one way to learn more about how these planets form is to study the disk of gas and dust from which they form.
Join the Boise State Physics Department on Friday, Oct 6 at 7:30p in the Multi-Purpose Classroom Building, room 101 to hear Prof. Hannah Jang-Condell of the University of Wyoming discuss her cutting-edge research on these protoplanetary disks and how the telescopes at University of Wyoming are being used to better understand and characterize exoplanets.
At 8:30p after the presentation, we will stargaze on the roof of the Brady Street Parking Garage, weather permitting.
The event is free and open to the public.
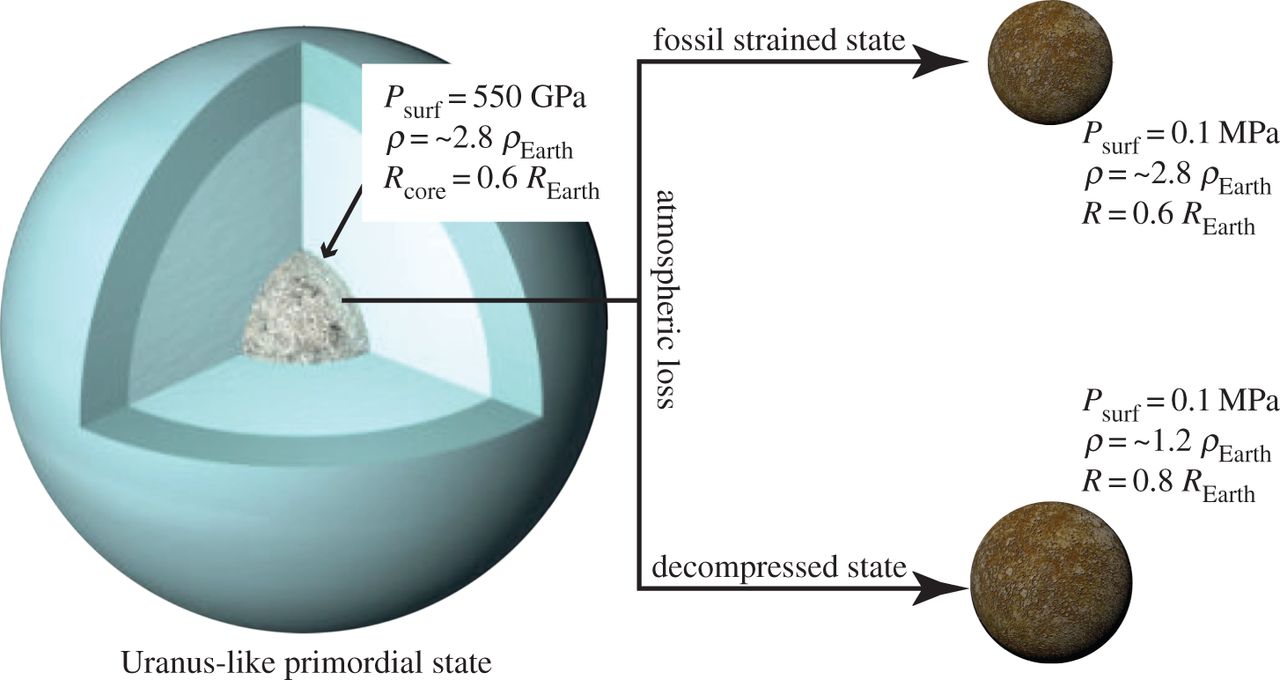
Figure from Mocquet et al. (2014) show how a gaseous planet might evolve into a dense, rocky core.
Another blast from the past, Mocquet et al. (2014) was the topic of our journal club this week, a paper that seeks to answer the question “What would Jupiter look like if you took away its atmosphere?”.
Given the enormous number of gas-rich exoplanets very close to their host stars discovered in recent years, many astronomers (including myself) have wondered whether such planets could have their atmospheres completely removed.
We certainly see some very hot planets where intense sunlight is blasting away their atmospheres, and in other cases, the star’s gravity can rip off the atmosphere. And so it’s not crazy to think some gaseous planets might completely lose their atmospheres.
Would anything be left over? Astronomers think that gas giants like Jupiter are like big cherries, with a squishy outer layer of gas wrapped around a dense pit of rock. Indeed, the Juno mission currently in orbit around Jupiter is designed to measure the size of Jupiter’s core by measuring its gravitational field very precisely.
And so the cores of gas giants are under enormous pressure – for instance, the core of Jupiter is being squeezed by 45,000 times the pressure at the bottom of the Mariana Trench on Earth.
In their study, Mocquet and colleagues explore what happens to a rocky core under such large pressures. Not surprisingly, they find that such a core would have an enormous density, perhaps three times larger than the Earth’s.
But what is surprisingly is that their results suggest the core might retain a very large density even if you removed the overlying atmosphere. It’s as if you squeezed down a nerf ball and then let it go – instead of springing back immediately, the nerf ball would take a few billion years to decompress. This means that we might be able to identify the cores of former gas giants by looking for planets roughly the size of Earth but with anomalously high densities.
And in fact, such planets have been found – the planet Kepler-57 b has a mass more than 100 times Earth’s but squeezed into a volume only ten times larger, giving a density of almost 44 grams per cubic centimeter – twice the density of the densest element on Earth, osmium.
So in their search for fossils, gas giant paleontologists should keep in mind that the bones of extinct gas giants may have distinctively large densities, almost as dense as adamantium.
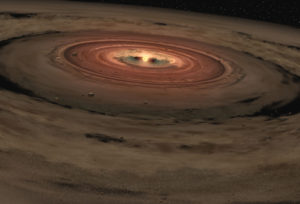
Artist’s conception of a protoplanetary disk from which planets form.
During today’s research group meeting, we discussed a paper from a few years ago from Lars Buchhave and colleagues that investigated the relationship between the composition of a planet-hosting star and the properties of its planets.
The discoveries of thousands of exoplanetary systems in the last few decades has revealed the bewildering variety of planets formed in our galaxy, and the richness of this planetary zoo probably reflects the wide range of conditions in which these planets formed.
Going back to the philosopher Kant, planets have been thought to form in disks of gas and dust leftover after their host star forms, and we now have a plethora of observational and theoretical evidence supporting this idea.
This idea means that the star and planets form mostly from the same source of material. However, while stars form directly out of the disk, the formation process for planets is a little pickier about what goes into the planets.
For example, the Sun is made almost entirely out of hydrogen and helium, elements that constitute most of the baryonic matter in the universe, while the Earth is made mostly of rocky elements, which are pretty rare in the universe. The gas giant Jupiter is kind of a mix – it’s mostly hydrogen and helium like the Sun, but it has more of the heavier elements than the Sun, all of which astronomers refer to as metals.
In their paper, Bucchave and colleagues report estimates of the ‘metallicities‘ or the amount of metals in lots of planet-hosting stars and try to figure if the type of planets around a star depends somehow on stellar metallicity.
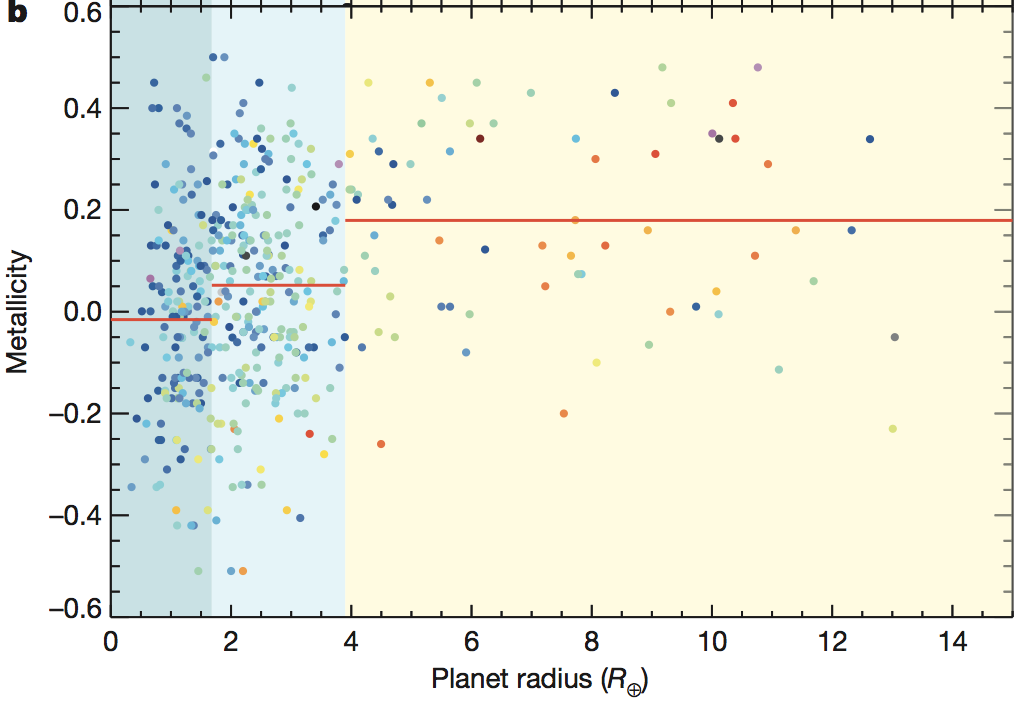
Figure 1 from Bucchave et al. (2014) shows the metallicities of stars vs. the radii (in Earth radii) of their planets. The horizontal red lines show the average metallicity for stars in that group.
Interestingly, the metallicities suggests there are three kinds of planetary systems – shown as dark blue, light blue, and yellow in the figure above. Big gaseous planets like Jupiter, with radii many times Earth’s, seem to form preferentially around stars with lots of metals, while small planets like the Earth aren’t as picky – they’ll form around stars with any metallicity. And planets with radii in between, about 2 to 4 times the Earth’s radius, they’re like Goldilocks and prefer stars with a little more metals but not too much.
What does all this mean? Astronomers think the protoplanetary disk (and therefore the star) might be required to have lots of planet-forming materials (that is, metals) in order to make big planets like Jupiter. On the other hand, forming small planets like the Earth apparently doesn’t take much because even stars with a tenth the Sun’s metals host them. Which all sort of makes sense.
But these results don’t answer everything. Why, for example, aren’t the stars with really big metallicities (the blue dots near the top left of the figure) always able to form big, Jupiter-like planets? This cluster of three blue dots are all members of the KOI-3083 planet system, whose star is Sun-sized but has almost three times more metals, but all the planets are smaller than Earth.
Could there be big planets in that system we haven’t found yet? Or maybe the planet formation process involves so much randomness (stochasticity) that a big metallicity only steers the system in the direction of big planets; it doesn’t force them in that direction. Like gently shepherding a toddler through a toy store – more often than not, you’ll end up with toys in your cart.
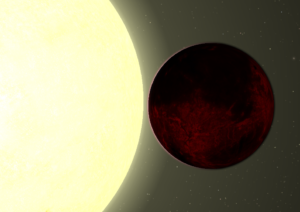
Artist’s conception of the ultra-short-period planet Kepler-78 b, discovered by Sanchis-Ojeda and colleagues in 2013.
An eyebrow-raising paper emerged recently from Prof. Josh Winn and colleagues about a type of planet near and dear to my heart, ultra-short-period planets, or USPs for short.
These planets are roughly the size of Earth and probably rocky but are hundreds of times closer to their host star than the Earth is to the Sun. These planets are so hot some have melted daysides and others are evaporating. Because they’re so much closer to their stars, ultra-short-period planets zip around their stars in just hours – hence the clunky name.
Our group, along with others, has suggested USPs might be the remnants of hot Jupiters (gas-giant planets close to their stars) that had their atmospheres ripped off. If so, we’d expect systems hosting USPs to resemble systems hosting hot Jupiters.
One distinctive feature of stars with hot Jupiters is that they have more iron (Fe) and other heavy elements in their atmospheres. Astronomers call the amount of heavy elements (“metals”) stellar metallicity. Hot-Jupiter host stars are heavy in metals probably because planets form from the same materials as the star and big planets need large amounts of metals to form. The same trend doesn’t seem to hold for small, roughly Earth-sized planets, though – small planets don’t seem to be as picky. So, if USPs are hot Jupiters that lost their atmospheres, their stars should also be metal-rich.
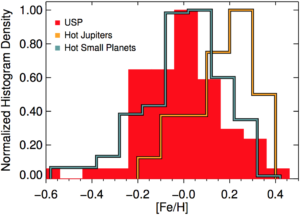
Figure 4 from Winn et al. (2017), showing the distribution of stellar metallicities for USP-hosting stars (red), hot Jupiter-hosting stars (orange), and stars hosting small but slightly longer period planets (blue).
But the recent paper from Winn and colleagues throws this origin story for USPs into doubt. In their study, they looked at metallicities for stars hosting USPs, stars hosting hot Jupiters, and those hosting small planets a bit farther out than USPs, all discovered by the Kepler Mission. The figure at left shows their results.
As expected, the orange curve for hot Jupiter hosts peaks toward higher metallicity (that is, toward bigger [Fe/H]-values), and if USPs are former hot Jupiters, the red histogram should look like the orange one.
Instead, it looks a lot like the blue one for smaller, farther out planets. This result suggests that USPs are just like their longer-period cousins – planets that have always been small, just with very short periods.
What to make of this? There’s some statistical wiggle room, allowing some, but not all, USPs to have been hot Jupiters, but Winn’s analysis says no more than 46%. It’s also possible that the boundaries between what Winn calls “hot Jupiters” and what he calls “hot small planets” could be refined by additional analysis, shifting the orange curve down a bit (or maybe shifting the blue curve up).
But the chances that USPs experienced a dramatic and brutal origin are a little slimmer now. Maybe that’s a good thing – it says the universe might be a little bit less violent than we thought.
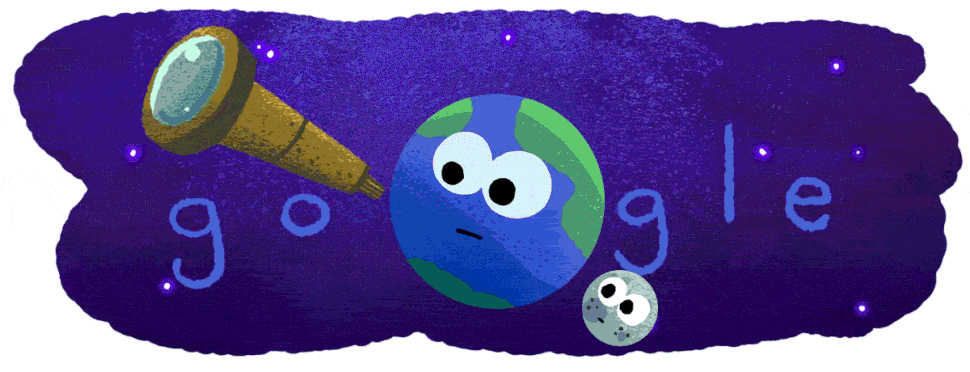
Google’s TRAPPIST-1 doodle.
UPDATE: KBSX ran a story about our event this morning – http://boisestatepublicradio.org/post/bsu-and-university-washington-part-search-life-nearby-planets.
The seven Earth-sized planets orbiting the nearby star TRAPPIST-1 reveal that rocky worlds are common in our galactic neighborhood. Three of the planets are in the habitable zone, the region around a star in which liquid water is possible. However, planets that are Earth-sized and in the habitable zone have merely cleared the first two hurdles for a planet to support life!
Join the Boise State Physics Department and Prof. Rory Barnes from University of Washington on Friday, April 7 at 7:30p in the Multi-Purpose Classroom Building, room 101 to learn about how these planets were discovered, what it means to us, and the potential of discovering life beyond our Solar System.
Contact Prof. Brian Jackson (bjackson@boisestate.edu) with any questions.
I just arrived home after a week-long visit to Aspen, CO to attend the Formation and Dynamical Evolution of Exoplanets Conference at the Aspen Center for Physics.
This conference was a cozy affair, with just over 100 attendees, and was narrowly focused on dynamical questions and approaches related to the origins and fates of exoplanet systems.
Researchers from around the world gave presentations on topics ranging from the dynamics of debris disks to observations of planet-hosting binary star systems. Blocks of presentations were punctuated by lengthy coffee breaks, when the real scientific give-and-take takes place. These interludes often give rise to groundbreaking, thesis-motivating, all-nighter-pulling research ideas.
Most of the presentations and conversations were excellent and inspiring, and I can’t do them all justice in a short blog post. So I’ll just talk about one that struck me in particular.
On Tuesday, Hanno Rein at Toronto spoke about a new N-body integrator his team has been developing in recent years, called REBOUND. This new framework may spur a revolution in dynamical modeling of astrophysical systems.
In astronomy, “n-body integration” is jargon for the numerical simulation of interactions among multiple (“n” of them) gravitating bodies. For hundreds of years, astronomers have been able to describe the orbital of two gravitating bodies quite easily, thanks to Johannes Kepler.
But as soon as you add another body to the system, there is no exact way to solve for the orbital motion of the bodies (except in very specific and limited circumstances). Even in the case of two bodies, if you want to include more complicated forces than simple gravity, solving for the orbital motion can be quite difficult.
To surmount these difficulties, scientists have turned to computer simulations to model in an approximate way the evolution of n-body systems. Although scientists have spent decades coming up with better and better models and algorithms, n-body simulations can still take a lot of computing power, and the often complicated codes can be cumbersome to set up and run. More than that, it’s often difficult or impossible for scientists to share results because there’s no good agreed-upon format for simulation output.
Rein’s REBOUND open-source code solves several of these problems at once: it employs latest modeling schemes to track orbital motions and gravitational interactions; it can be run using inside of an iPython Notebook; and it provides a uniform format for simulation output which anyone can use to re-run or re-analyze another scientists work – critical for scientific reproducibility. The iPython Notebook also provides a really neat visualization capability so you can directly watch the evolution of your astronomical system.
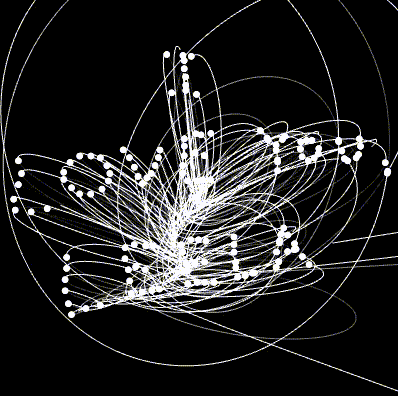
Time evolution of the orbits of stars in Leela’s constellation.
The code is so easy to run, in fact, that I installed and began running it immediately after Rein’s presentation. And all of its capabilities allowed me to finally simulate and visualize the evolution of a system I’ve wanted to look at for a long time – see animation at left (see here for how I created it).
I also gave a presentation on our group’s work looking at disruption of gaseous exoplanets.
And so, the combination of beautiful scenery and beautiful science made the Aspen Exoplanets conference one of the best in recent memory.