White dwarf stars have been a mystery since they were first discovered. Extraordinarily hot and compact, the engima of white dwarfs was unraveled largely through the herculean efforts of the Harvard computers. Astronomers now know white dwarfs are the final stage of a violent aging process for Sun-like stars. And though astronomers originally expected such violence would spell doom for any planets in orbit, mounting discoveries show that some planets at least can survive this cataclysmic descent into stellar senescence. Whether any life survives on those planets is another matter.
Too Hot to Handle
Our current understanding of stars owes an enormous debt to the computers at Harvard College Observatory operating during the late 1800s/early 1900s. Unlike the computers at Harvard today, though, these computers were living, breathing people, mostly women, who performed the complex and often tedious processing of astronomical and astrophysical data. (Indeed, there’s a long history of contribution by women to astronomical calculation.)
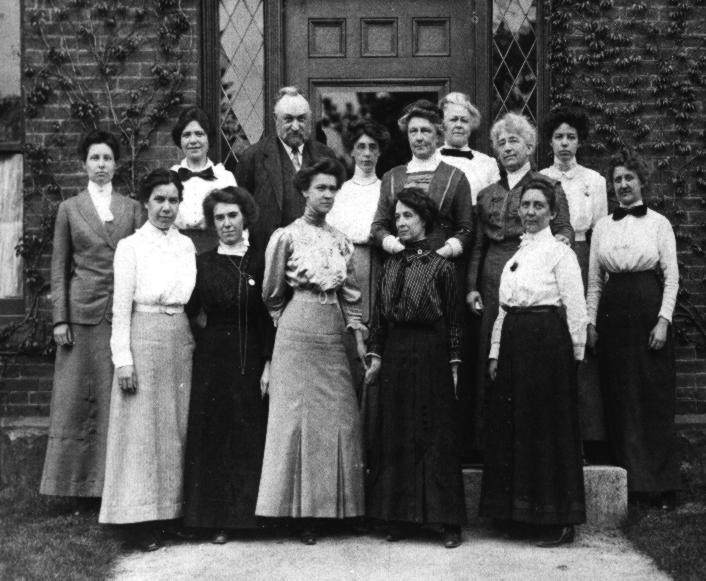
Among other tasks, the Harvard computers analyzed and cataloged hundreds of thousands of stellar spectra collected as part of the Henry Draper Stellar Catalog project, led by the preeminent astronomer Edward Pickering. As spectra piled up, subtle but profound patterns emerged, bespeaking the natures and origins of the distant stars.
By the ninenteen-teens, one of the most important patterns had crystallized: for most stars, the hotter they were, the more luminous they were. They very hottest stars (which were usually the brightest) were labeled class A, while the coolest (usually the dimmest) were class M (don’t ask why). Though, at the time, the reasons for this relationship were unclear, today, astronomers understand it represents the most stable phase of a star’s life. Stars in this phase are said to be on the main sequence.
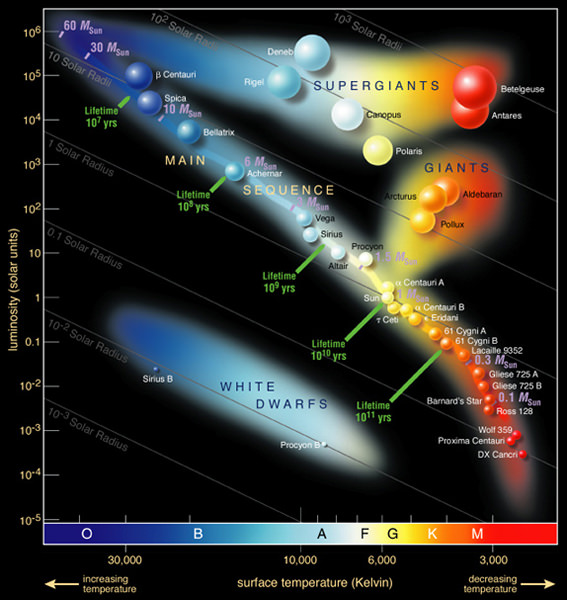
But not all stars followed this pattern. In fact, some of the most well-known stars seemed to be misbehaving, a tale told by one of the pioneers of stellar evolution theory, Henry Russell Norris:
[In 1910, ] I was visiting my friend and generous benefactor, Prof. Edward C. Pickering. … I asked Pickering about certain other faint stars, … mentioning in particular 40 Eridani B. [H]e sent a note to the Observatory office and before long the answer came (I think from Mrs. Fleming) that the spectrum of this star was [class] A. I knew enough about it, even in these paleozoic days, to realize at once that there was an extreme inconsistency….
Schatzman, E. (1958). White Dwarfs.
As class A, 40 Eridani B has a temperature approaching 10,000 degrees Kelvin (18,000 degrees Fahrenheit) but is more than 25,000 times dimmer than class A stars on the main sequence!
Astronomers now know such blistering but dim stars are white dwarfs. As a star ages, eventually the nuclear fusion that power it shuts off, sending the star off the main sequence, and what we see as a white dwarf is simply the cooling extinct nuclear furnace from the star’s center. Their small sizes (some pack a Sun’s worth of mass into the volume of Earth) is what makes them so dim.
But even though white dwarfs lack the luminous punch of their main sequence rivals, they can still exert a substantial gravitational influence and can, as it turns out, hold onto planets.
The (Gravitational) Pull of Distant Worlds
A recent study from Tasmanian astronomy Joshua Blackman and colleagues found just such a planetary system and leveraged the unique properties of white dwarfs to do it.
The system, unpoetically named MOA-2010-BLG-477L, was originally discovered by Dr. Etienne Bachelet and colleagues using a technique called gravitational microlensing. The figure below shows how that technique works, but by way of a brief explanation:

One star, called the “lensing star” passes almost in front of another, background star, the “source star” (as seen from Earth). As the lensing star sweeps past the lensed star, its gravity redirects some of the light from the source star. This “lensing event” makes the source star suddenly appear much brighter than before. If the lensing star also hosts a planet, the brightening signal will show multiple peaks, one for the star and one for the planet. That’s just what astronomers saw for MOA-2010-BLG-477L, and the size and timing of the planetary peak showed it was due to a Jupiter-like planet orbiting at least twice as far from its star as the Earth.
After lensing, MOA-2010-BLG-477L continued on its lonely course around the Milky Way. Astronomers expected that it should emerge from the glare of the lensed star, allowing them to measure how bright MOA-2010-BLG-477L. That’s where Blackman and his team came in. They used the 10-meter wide Keck-II telescope to catch a glimpse of the system. The figure below shows what they saw, or, rather, what they didn’t see: MOA-2010-BLG-477L was nowhere to be found.
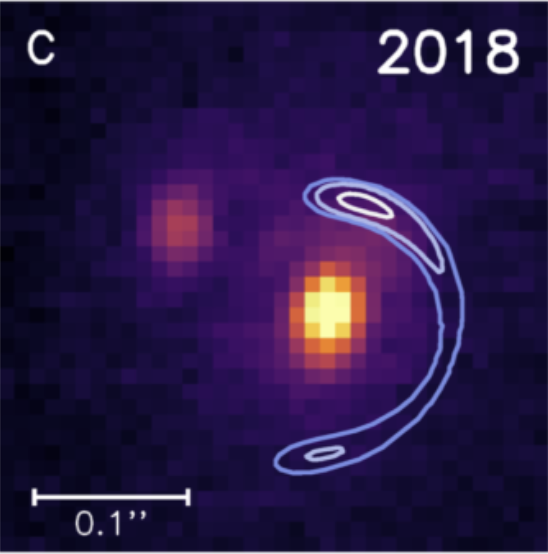
Since they didn’t see MOA-2010-BLG-477L, they could only conclude that the star must be very dim. The only kind of star dim enough not to be seen but also massive enough to produce the microlensing curve is a white dwarf star, making MOA-2010-BLG-477L the only one known to host a Jupiter-analog planet.
Planetary systems orbiting dead stars are now known to be, if not ubiquitous, at least not uncommon. In fact, the very first exoplanets discovered orbit an exotic type of star that aged off the main sequence, the pulsar PSR B1257+12.
Though the three planets orbiting PSR B1257+12 are roughly Earth-like, they are very unlikely to host life: one hundred sixty-one times a second, the pulsar fires its beam of high-energy radiation at the worlds, completely sterilizing them.
MOA-2010-BLG-477L is only a quiescent white dwarf and not a deathbeam-wielding pulsar, but any life in that may have existed in that system was probably wiped out long ago.
Worlds of Future Past
If you’ve watched the Star Trek: TNG episode, “The Inner Light“, you know that the process by which main sequence stars become white dwarfs is anything but gentle. After billions of years steadily burning on the main sequence, stars like the predecessor of MOA-2010-BLG-477L become unstable as their internal nuclear furnace begins to sputter. Eventually, the star’s outer envelope expands, making the star into a red giant and bathing any nearby planets in molten plasma. This outer envelope can escape from the remaining stellar core, leaving behind a white dwarf.
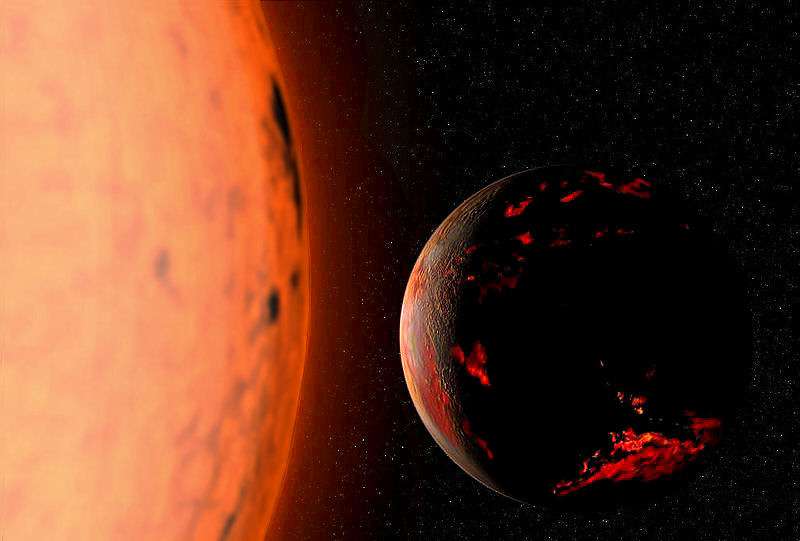
That’s why a system like MOA-2010-BLG-477L is so surprising to find. Before such discoveries, astronomers expected that the red giant phase of stellar evolution would spell doom for a planetary system. But apparently, some planets can survive.
What about our own solar system? In about five billion years, our Sun will undergo its own red giant phase, puffing out its atmosphere and very likely consuming Mercury and Venus in the process. Stellar evolution models don’t yet have sufficient resolution to tell us whether that plasma bubble will reach the Earth.
But as far as terrestrial life is concerned, it won’t matter. The enormous increase in luminosity that accompanies the Sun’s metamorphosis will vaporize the oceans and sterilize our planet. The only hope for terrestrial life at that point is to find another planet in a distant solar system, a prospect that will bring its own perils.
If you want to learn more about the fate of white dwarfs and the long-term evolution of the Universe, check out The Five Ages of the Universe: Inside the Physics of Eternity by astronomers Fred Adams and Greg Laughlin.