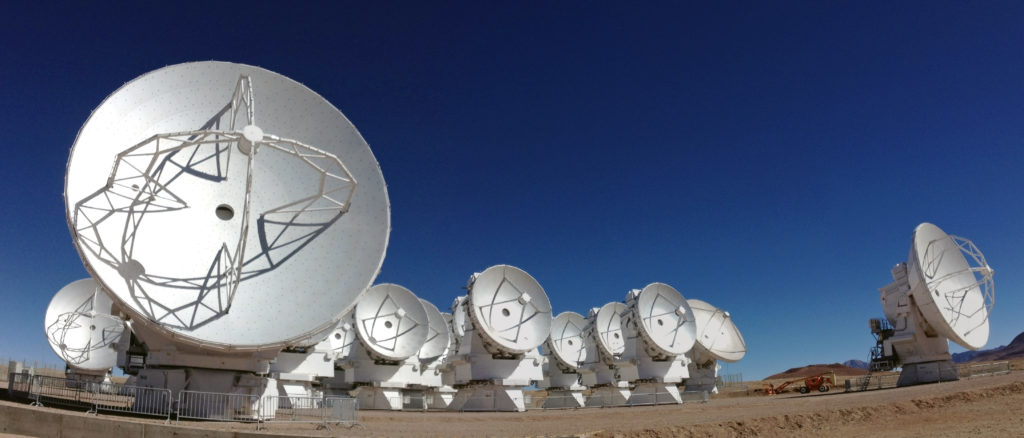
Atacama Compact Array (ACA) on the ALMA high site at an altitude of 5000 metres in northern Chile. From here.
If you haven’t heard about it, the ALMA array, a collection of sixty-six, 12-meter radio dishes situated high in the Atacama desert, is phenomenal. Using the technique of radio interferometry, it’s capable of imaging astronomical objects in infrared (and redder) light with incredibly high resolution.
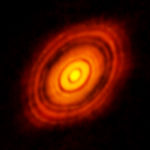
HL Tauri, as seen by ALMA.
For instance, the image at left was captured by ALMA and shows the debris disk in an infant planetary system orbiting a distant star, HL Tauri. The bull’s-eye pattern is (probably) created by nascent planets still growing by scooping up dust and gas. That disk is physically larger than our whole solar system, but as seen from Earth, 450 light-years away, the disk subtends an angle about 3 micro-degrees across – about the same as the Statue of Liberty as seen from Boise.
As it turns out, Jupiter’s moon Europa, an icy body only a little smaller than our moon, is about as big seen from Earth, making a good target for the ALMA array. Moreover, since the Galileo mission‘s exploration of the Jupiter system, few detailed and high-resolution observations have been made of Europa. On top of that, Europa has a subsurface water ocean that could host alien life.
With all this in mind, Caltech graduate student Samantha Trumbo and Prof. Mike Brown (of Pluto-killing fame) collected ALMA observations of Europa in the fall of 2015. Since ALMA observes in infrared wavelengths, it’s sensitive to heat coming off Europa and essentially acts as a long-distance thermometer, allowing them to map the temperature on Europa’s surface. If certain parts of Europa are warmer than expected, that could indicate sub-surface heating, which might have big implications for any Europan life.
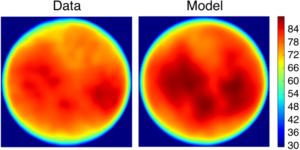
Temperature map of Europa, from Trumbo et al. (2018).
But instead of mysterious hotspots, Trumbo found equally strange cold spots. The color map of Europa at left (red means hot, blue means cold) compares the expected temperatures (“Model”) to what’s actually observed (“Data”), and there are big differences all across Europa.
So what does this mean? Trumbo et al. say it’s not clear but suggest one possibility. The region with the largest temperature discrepancy corresponds to the location of highest water ice abundance, where water from the sub-surface may have been volcanically extruded onto the surface. Since this region was not been imaged at high resolution by Galileo, it’s hard to identify landforms that might corroborate recent eruptions, but such features have been observed elsewhere on Europa.
As always in science, more data would help resolve the puzzle. In any case, NASA is planning a mission for launch in the 2020s that will use an ice-penetrating radar, not too different from ALMA, to probe Europa’s sub-surface ocean and, hopefully resolve the mystery of Europa’s cold spots.