Today we had a great DTM seminar by Rachel Osten from the Space Telescope Science Institute (STScI). She talked about stellar activity and coronal mass ejections (CMEs).
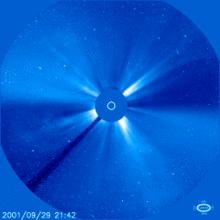
A coronal mass ejection in time-lapse imagery obtained with the LASCO instrument. The Sun (center) is obscured by the coronagraph’s mask.
Osten pointed out that these huge eruptions from stars are important for several reasons. For example, the energy and frequency of CMEs depend on several properties of stars, including their age, rotation rates, and magnetic fields. In general, as stars age, their rotation rates drop, usually reducing the strength of their magnetic fields and the amount of CME activity. And so learning about CMEs can tell us about stellar evolution.
When a star fires off a CME, it typically flares or brightens a bit (see figure at right), and since we can’t see stars other than our Sun up close, we can use the temporary brightening of those stars to study their flare activity. Osten talked about one of her projects to use data from the Hubble Space Telescope to look for flare activity for many stars near the constellation Sagittarius.
That project had a surprising result: many stars that were thought to be older than the Sun actually showed MORE flare activity than they should have. This result might mean these stars actually have previously unknown binary companions that kept the stars spinning quickly and thereby keeping their flare activity up.
Stellar flares may also be important for planetary habitability. For example, a big stellar flare can actually disrupt the atmosphere of an Earth-like planet, perhaps even removing all of the life-protecting ozone.